Emerging Plastics Recycling Technologies: Where Are They Headed? Part 1
Plastics have multiple applications, and they are cheap to produce. But the explosion in manufacturing and consumption far outstrips the capacity to manage them at the end of life. The flow of plastic into the ocean is projected to nearly triple by 2040, and the current methods of handling the waste comes with steep expenses that overshadow the low cost of virgin plastics, says The Pew Charitable Trusts.
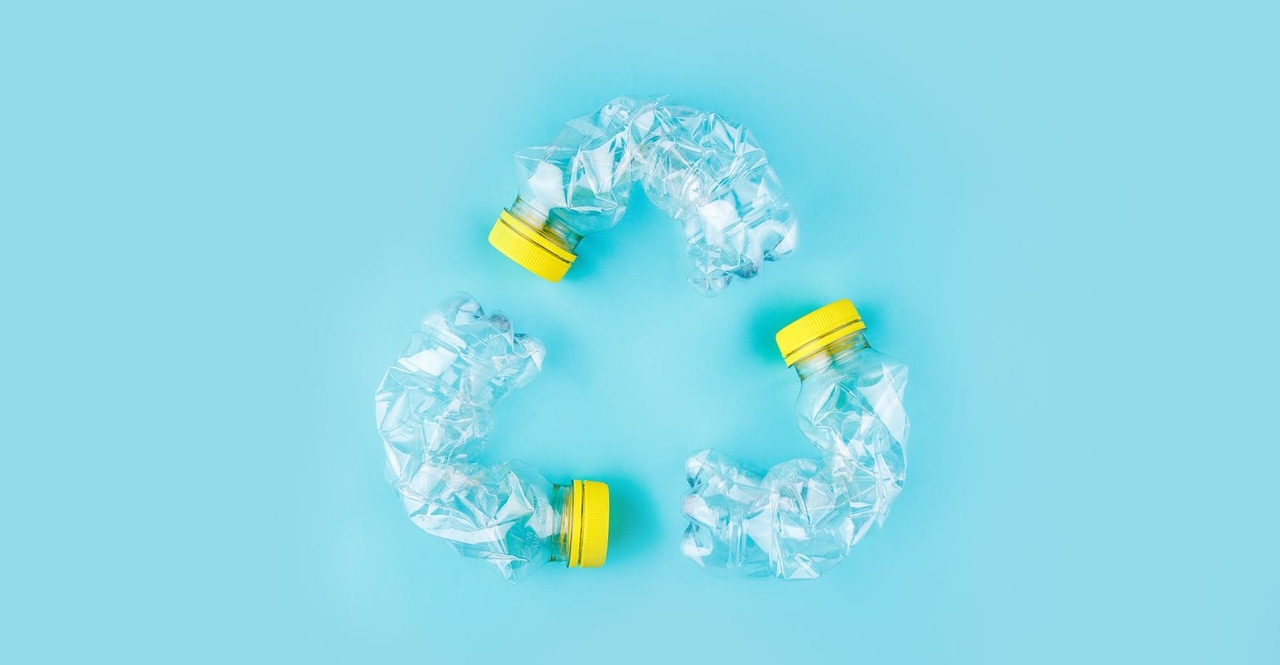
This two-part series assesses several emerging recycling technologies, including chemical processes and an experimental biological process, as well as a few more established mechanical recycling methods. It goes on to discuss what some industry experts say is needed in the way of policy and funding to advance the emerging technologies.
Plastics have multiple applications, and they are cheap to produce. But the explosion in manufacturing and consumption far outstrips the capacity to manage them at the end of life. The flow of plastic into the ocean is projected to nearly triple by 2040, and the current methods of handling the waste comes with steep expenses that overshadow the low cost of virgin plastics, says The Pew Charitable Trusts.
Policymakers, consumers, and some brands and manufacturers –all aware of this problem –are driving a trend: the rethinking of the world’s approach to plastic, including ways to recycle it. New technologies, and tweaks to old technologies, are evolving aimed at improving recovery and processing of these materials to keep them out of the environment, and in circulation at their highest use.
Economist Impact, the research arm of the Economist Group, which reports on global political and economic affairs, has released a study: Rethinking Plastics in a Circular Economy, funded by Dow Chemical that assesses several recycling technologies under the categories of chemical and biological approaches (often called “advanced recycling) as well as some mechanical techniques.
Economist Impact’s assessment is broken down into five categories:
Applicability: Number of plastic types the technology can process; ability to manage multi-material or mixed waste streams; and level of sorting and decontamination required.
Quality of output: Whether recycled output is of similar or higher quality or lower quality than the input, and whether the output is food-grade.
Efficiency & sustainability: Looking at process temperatures and whether the process is ‘open loop’ (output cannot be used to produce the original product) or ‘closed loop’ (output can be used to produce the original product).
Integration: How well the method integrates with downstream processes throughout the entire waste management system, and range of applications of output.
Reach & scalability: Maturity; the number of operational and planned facilities; and their capacity.
The chemical processes explored are:
Catalytic pyrolysis (thermal decomposition of plastic waste in the absence of oxygen using a catalyst to enhance varied performance metrics).
Non-catalytic thermal pyrolysis (thermal decomposition of material in the absence of oxygen to produce a plastic oil).
Plasma pyrolysis [integrating conventional pyrolysis with thermochemical properties of plasma to transform plastic waste into synthesis gas (syngas)].
Gasification (involving heat and high pressure with little to no oxygen to produce syngas that can be converted into monomers).
Chemolysis (using chemical agents or just water to break down plastic into monomers).
Hydrothermal recycling (using water, high pressure, and high temperature to cut hydrocarbon bonds in plastics to make oils and chemicals and ultimately to make new plastics).
Also examined was a biological process:
Enzymatic hydrolysis (using an enzyme that dwells in waste that is combined with another enzyme to accelerate breakdown of plastics into monomers).
Also assessed were these mechanical processes:
Melt filtration and extrusion (melting plastic to filter out impurities and passing it through an extruder to make plastic pellets).
Flake-to-preform (making preforms by flaking, decontaminating, melting, filtering, then feeding the material into an injection molding machine, skipping pelletization).
Purification/dissolution (dissolving plastic in a solvent, separating the polymer from additives and contaminants, then crystalizing the polymers (referred to by others in the industry as physical and/or chemical recycling rather than mechanical).
Martin Koehring, director of the research for this study says, “There is a huge divergence between these technologies, and they come with tradeoffs, which is why this study is so important. It comprehensively assesses each plastic recycling technology for attributes that will be important considerations to policymakers, investors, the plastics industry, and other stakeholders who will be key to advancing these technologies.”
Some of the main takeaways are that the chemical processes, especially pyrolysis, gasification, and hydrothermal recycling, produce high-quality, high-value outputs. And they can work with multi-material packaging, flexible packaging, and mixed waste streams.
All the chemical technologies scored high for their ability to process food-grade materials (flake-to-preform was the one mechanical process that also performed well in this area). Enzymatic hydrolysis also is effective with food-grade and leads the way in opportunities for upcycling, delivering a product of similar or higher value than in its first life.
A drawback of the advanced processes, notably pyrolysis, gasification, and hydrothermal recycling, is that they are energy-intensive and can generate carbon dioxide emissions unless powered by renewable energy.
Additionally, many of them depend on chemical reactions that present potential safety hazards. So, they require high investments in engineering and construction as well as advanced safety protocols to mitigate associated risks.
And with the chemical processes the recycled output will require a lot of preparation to produce new plastic materials.
Study interviewee Suhas Dixit, founder and chief executive of APChemi, said, “You cannot just take plastic oil [after pyrolysis] and start feeding it into a cracker.” APChemi builds conventional thermal pyrolysis recycling plants globally.
Chemolyis, however, was an exception, found to need little preparation prior to production compared with pyrolysis and gasification. Though Koehring projects it will be commercially viable only on a large scale.
Of note, he says, “With many chemical technologies, and the biological process we assessed there are questions around technical maturity and operational capacity. Many of these advanced recycling technologies are not proven at large scale, and the path to commercialization is not there, while most of the mechanical technologies we examined are commercially viable, with high operational capacity.”
Of the “advanced” processes, gasification, catalytic and non-catalytic pyrolysis, and hydrothermal technologies are the furthest along; they’re at early commercial installation stages; a few are already operating on a commercial scale. Chemolysis is at the demo stage. And enzymatic hydrolysis and plasma pyrolysis are still at lab stage.
On the “downstream integration” front, the mechanical options were the strongest. They do well to integrate the recycled output into the next step in the value chain.
Flake-to-preform ranked highest in ability to close the loop (use material to make original product). But because it can usually only return materials to their original format this option is limited in terms of applicability to various materials or products.
Overall, the mechanical processes had a lesser quality output than advanced recycling methods, sometimes losing quality during processing, or at least unable to be upcycled into a higher quality product.
Looking ahead, chemical recycling technologies are well placed to complement mechanical recycling, Koehring says.
“Both have limitations and advantages. While advanced chemical technologies have potential to produce higher quality and higher value output, mechanical recycling will continue to be the primary path for some materials because they require fewer steps and are already established and commercially viable.”
Koehring emphasizes policies and industry initiatives are needed in order to implement and scale these innovations and strengthen the overall recycled plastic value chain. Stay tuned for Part 2 of this series for thought leaders’ ideas on what’s needed on the policy front, as well as other considerations, for scaling.
About the Author
You May Also Like